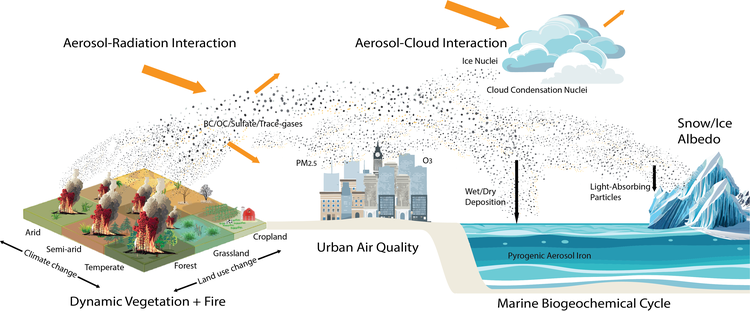
Interactive Fire Emissions in Earth System Models
This research is part of a continued effort to study the impacts of wildfires on the Earth’s climate systems and feedback. The GFDL Earth System Model (ESM4) solves for the carbon cycle with a dynamic land model (LM4) which also simulates wildfires. The next step will be to calculate wildfire emissions and injection heights from LM4 and emit aerosol and trace gases into the atmosphere (AM4) within the coupled modeling framework. Such a setup will provide a fully interactive model to study wildfire interactions within Earth’s climate system such as: Future projections of fire emissions and injection heights, future projections of atmospheric absorption, future projections of cloud properties, feedback on vegetation and fire dynamics, fires affecting atmospheric chemical composition under climate change, fire aerosols acting as light-absorbing particles, and wildfire-iron emissions affecting biogeochemical cycle.
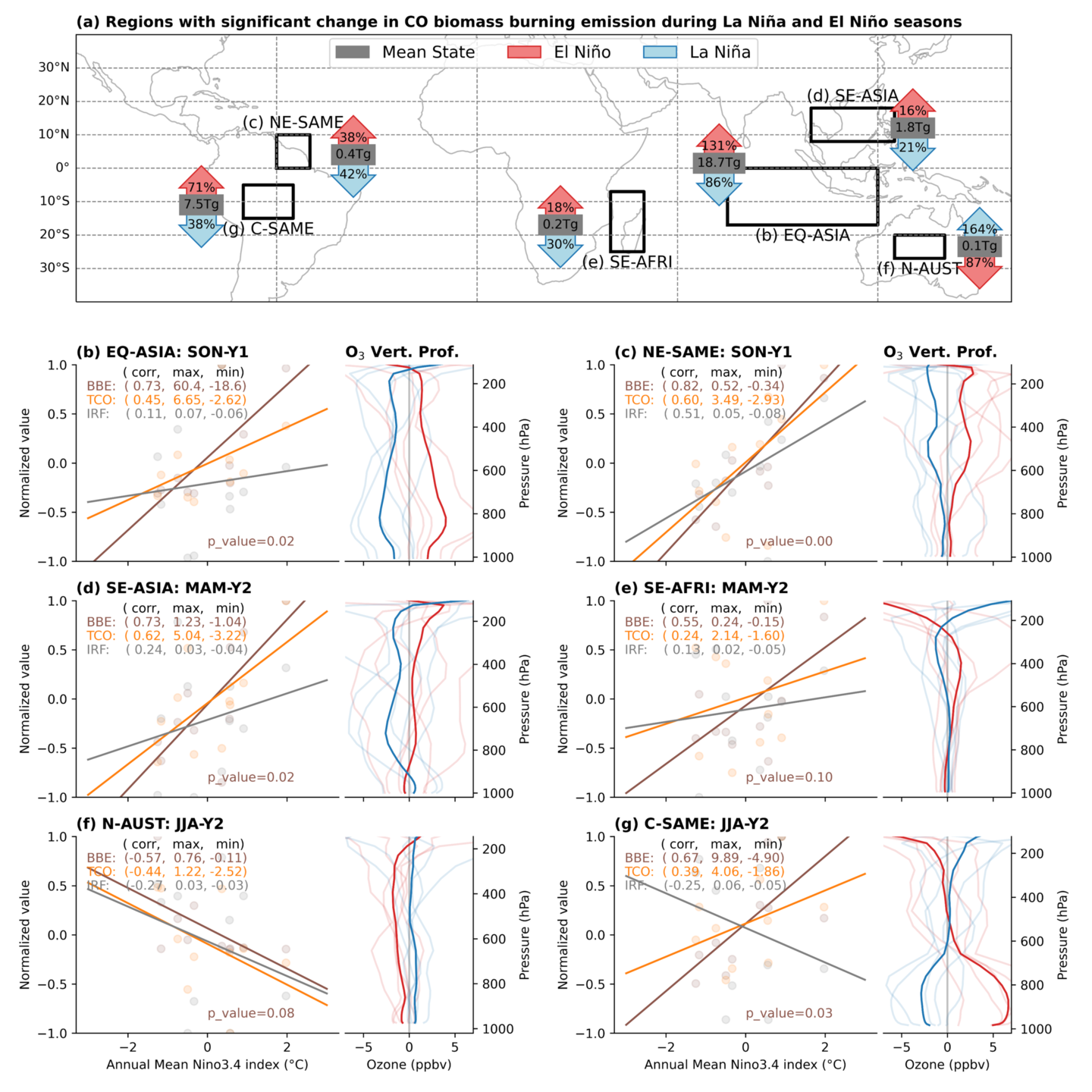
Instantaneous Radiative Forcing of Tropospheric Ozone
Understanding the natural drivers of tropospheric ozone variability (such as lightning, BB, and STE) is essential, as each of these factors significantly shapes the vertical ozone profile, influencing both ozone’s radiative effects and broader climate impacts. To this end, in this study, we employ a climate-chemistry model, and partial radiative perturbation (PRP) method to quantify the influence of natural drivers (lightning, BB, and STE) controlling the variability of tropospheric ozone radiative effects across different ENSO phases.
This plot shows the linear relationship between BB emissions, TCO, and TCO-IRF with the annual mean Nino3.4 index for regions where BB emissions exhibited significant changes (above 90% significance level) during La Niña to El Niño seasons. Despite the nonlinear chemistry of tropospheric ozone, we observed a distinct linear relationship in regions where increases in NOx/VOCs/CO emissions from biomass burning led to a corresponding rise in tropospheric ozone. In these regions, the available hydrocarbons adequately react with NOx to form ozone near surface and/or in upper troposphere by smoke plume-rise mechanisms or convective mixing. We identified a linear relationship that highlights the connection between increased ozone load, its vertical distribution, and tropospheric ozone radiative forcing.
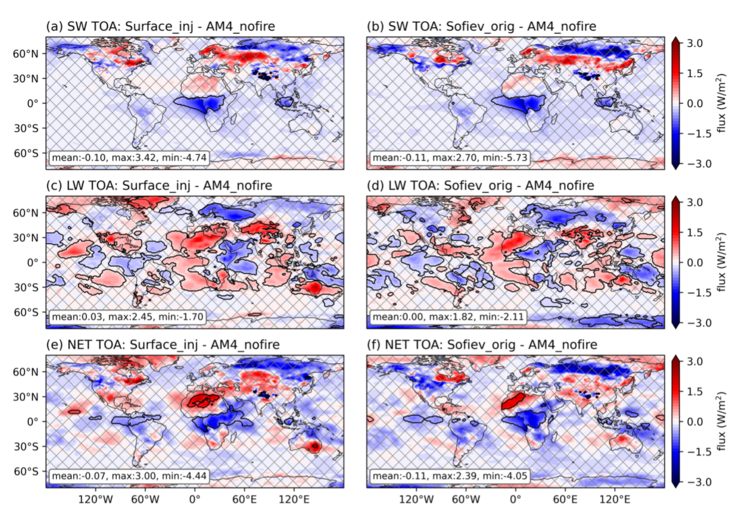
Dynamic Fire Injection Height in GFDL's AM4.0
Wildfires inject aerosols into the atmosphere at varying altitudes, modifying long-range transport, which impacts Earth’s climate system and air quality. Most global climate models use prescribed fixed-height injections, not accounting for the dynamic variability of wildfires. In this project, we enhance the injection method of biomass burning aerosols implemented in the Geophysical Fluid Dynamic Laboratory’s Atmospheric Model version 4.0, shifting to a more mechanistic approach. We test several injection height schemes to assess their impact on the Earth’s radiation budget by performing 18-year global simulations.
In summary, dynamic injection of biomass burning emissions changed the net clear-sky radiative flux at top of the atmosphere regionally (±1.5 Wm-2) and reduced it by -0.38 Wm-2 at the surface globally, relative to a baseline with no fire emissions. The temperature gradient anomaly associated with the dynamic injection of absorbing aerosols affects the atmospheric stability and circulation patterns. This study highlights the need to implement dynamic injection of fire emissions to simulate more accurately the atmospheric distribution of aerosols and their interactions with Earth’s climate system.
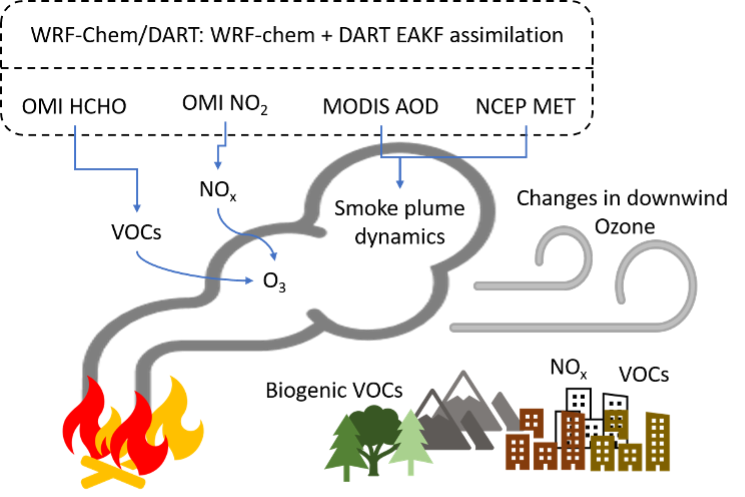
Downwind Ozone Changes from Fires
This study looks at how the Williams Flats wildfire in August 2019 affected ozone and its chemistry in areas downwind of the fire. We used satellite retrievals and weather measurements to study this and found that by assimilating satellite data on nitrogen dioxide (NO2) and formaldehyde (HCHO) columns, we could make better surface ozone predictions. We also used data on aerosol optical depth (AOD) to better simulate the movement of the wildfire smoke.
We found that areas closer to the fire had higher levels of nitrogen oxides, peroxyacetyl nitrate, nitric acid, and oxygenated volatile organic compounds, which contributed to an increase in ozone levels by 3-5 parts per billion in nearby areas and 2-3 parts per billion in areas further away. During more intense wildfire days, carbon monoxide and ozone plumes were transported over the Rocky Mountains to the east.
This figure shows how and why the data assimilation system helps us follow the wildfire plume's dynamics and chemistry. We use various observations to constrain chemistry and meteorology so that the transport of smoke plumes is accurately simulated, and we will eventually use the data assimilation model outputs to investigate ozone changes in the downwind region.